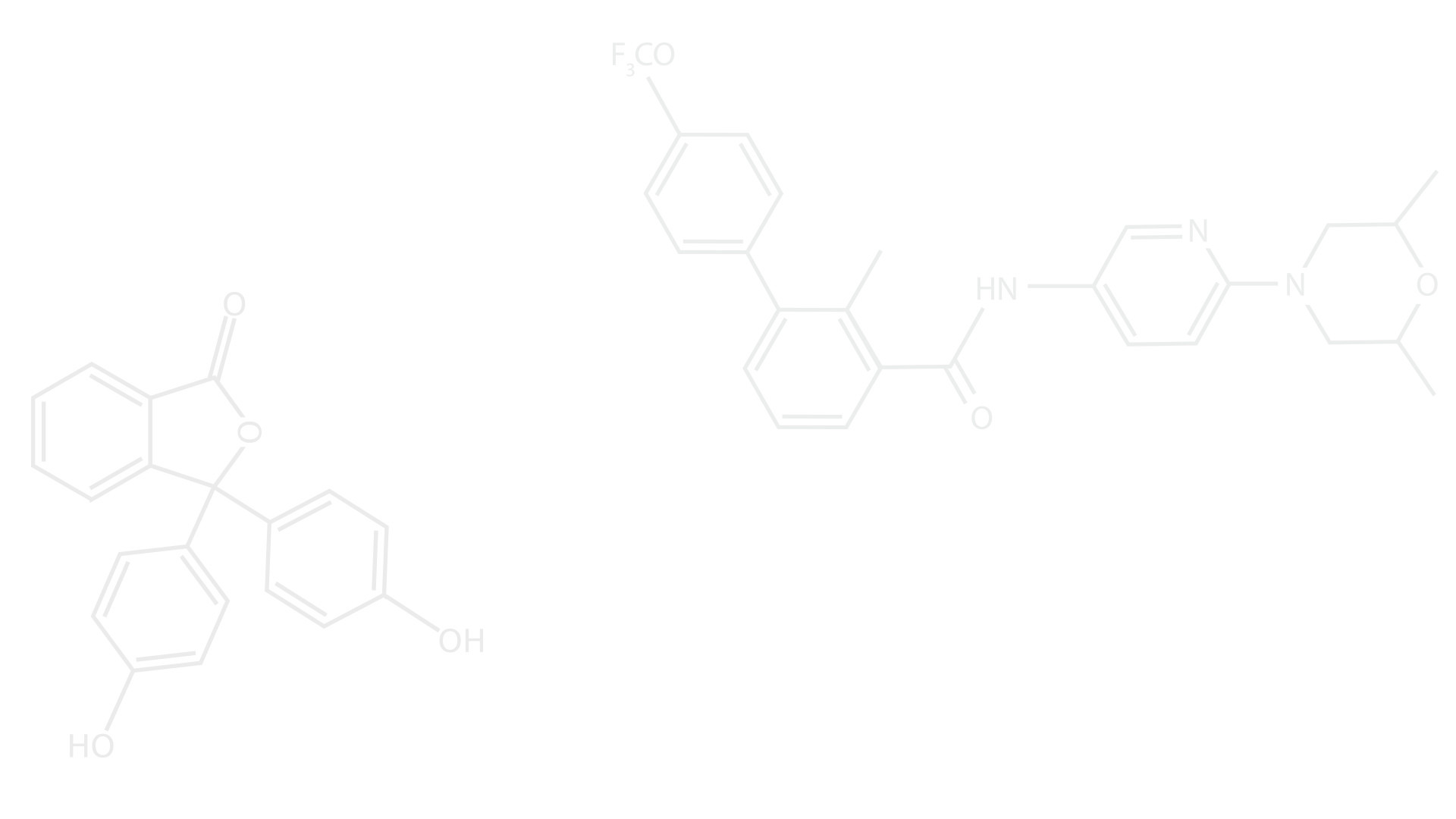
Preprint Club
A cross-institutional Journal Club Initiative
Poxvirus attack of anti-viral defense pathways unleashes an effector-triggered NF-κB response
BC Remick et al., (bioRxiv) DOI: 10.1101/2025.03.04.641538
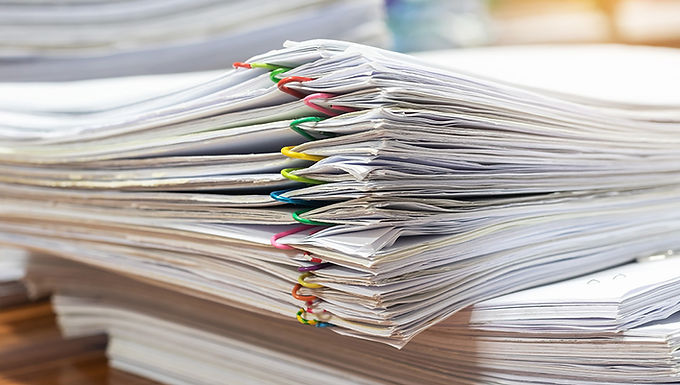
Keywords
Effector-triggered immunity
Myxoma virus
Human monocyte screening assay
Main Findings
Initial sensing of pathogens typically involves recognition by germline-encoded pattern recognition receptors (PRRs). Effector-triggered immunity (ETI) represents an additional layer of pathogen sensing, whereby host cells detect pathogen-induced perturbations pf cellular processes or structures caused by virulence factors (“effectors”).
To systematically identify ETI pathways in human cells, Remick et al. developed a screening approach in which individual Myxoma virus (MYXV) virulence factors are expressed in human B-lymphoma-derived monocytes (BLaER1 cells), followed by transcriptomic analysis via RNA-sequencing.
Using this platform, the authors identify and validate the MYXV effector M3.1 as a potent inducer of NF-κB signaling, independently of Toll-like receptor pathways. Mechanistically, M3.1 enhances viral replication by targeting antiviral proteins, including ZAP and TRIM25, and by inhibiting TBK1-mediated type I interferon (IFN) signaling.
Furthermore, the authors also show certain evidence that M3.1 suppresses two known negative regulators of NF-κB: N4BP1 and ZC3H12A. The inhibition of these regulators appears to unleash an ETI response characterized by NF-κB activation. While orthologs of M3.1 from related poxviruses (e.g., rabbit fibroma virus, swinepox virus, and lumpy skin disease virus) also activate NF-κB, MYXV infection itself does not significantly induce NF-κB–dependent IL-6 expression in BLaER1 monocytes, showing the complexity of viral-host interactions.
Limitations & Suggestions
Species relevance of the model:
Since MYXV is a virus adapted to rabbits, it would be valuable to investigate whether M3.1 also induces NF-κB signaling in rabbit-derived cells. This could clarify whether NF-κB activation by M3.1. is an adaptive feature selected in the natural host and whether it contributes meaningfully to viral replication.Lack of IL-6 induction by knock down of N4BP1:
In Figure 4, N4BP1 knockdown alone does not induce IL-6 in BLaER1 cells, nor does the additional M3.1 expression in the absence of N4BP1 lead to IL-6 induction. However, combined knockdown of ZC3H12A and N4BP1 results in IL-6 induction. This effect is not discussed in the main text, despite being a potentially critical insight into the functional interplay between these negative regulators. Does this suggest a threshold model for NF-κB activation? Or a form of redundancy in negative regulation?Use of poorly permissive cells to show MYXV induced NF-κB signaling: The authors show that MYXV replicates poorly in BLaER1 monocytes (Fig. S4), yet use these cells to assess IL-6 induction after viral infection (Fig. 5). Since no significant iL-6 induction is observed, it may be worthwhile to show the NF-κB activation by luminescence upon infection in a more permissive cell line, such as HEK293T, where MYXV replication is more robust. If there is no NF-κB in HEK293T cells, this could then be discussed. Also, it might be interesting to use another permissible cell line to support the result.
Supplementary Figure S1B shows a reduction in type I IFN response in M3.1-transduced cells, which fits to the TBK1-dependent suppression of IFNB1 later shown in Figure 3D. However, this connection is not mentioned in the main text and could help reinforce the proposed mechanism of TBK1 inhibition. A brief discussion of this link would strengthen the narrative.
Significance/Novelty
This study presents a novel screening approach to identify ETI responses in human monocytes and successfully uncovers M3.1 as a dual-function effector that both disables antiviral responses and induces compensatory NF-κB signaling. While the iinability of MYXV infection alone to induce NF-κB responses underscores the complexity of host-pathogen dynamics, the screening platform developed here could be applied to a wide range of pathogens, helping to uncover previously unrecognized mechanisms of innate immune sensing.
Credit
Reviewed by Lisabeth Pimenov-Reifeltshammer as part of a cross-institutional journal club between the Vanderbilt University Medical Center (VUMC), the Max-Delbrück Center Berlin, the Ragon Institute Boston (Mass General, MIT, Harvard), the Medical University of Vienna and other life science institutes in Vienna.
The author declares no conflict of interests in relation to their involvement in the review.